Oxidants versus Antioxidants: a Basic essay: Difference between revisions
Line 34: | Line 34: | ||
Hypochlorous acid (HClO), produced as an intermediate step through this reaction, plays a crucial role in immune response by combating bacteria, viruses, and other pathogens as well. | Hypochlorous acid (HClO), produced as an intermediate step through this reaction, plays a crucial role in immune response by combating bacteria, viruses, and other pathogens as well. | ||
== Glutathione == | |||
[[File:Gsh.png|thumb]] | |||
Glutathione, a crucial antioxidant found in human cells, plays a significant role in maintaining cellular health and protecting against oxidative stress. However, its availability is not limited to human physiology; bacteria also utilize glutathione to enhance their growth and proliferation. In microbial environments, glutathione acts as a key nutrient that facilitates various metabolic processes, '''enabling bacteria''' '''to thrive''' under the to high oxidative conditions Caused by OH radicals. While it is essential for the detoxification and repair mechanisms in human cells, the same properties allow bacteria to mitigate oxidative damage and promote their survival. This duality highlights the complex interplay between host and microbial populations, where glutathione serves as both a protective agent for human cells and a growth factor for bacteria. Understanding this relationship is vital for developing strategies to manage bacterial infections and maintain cellular integrity. | |||
In the study of microbial ecology, one can observe that certain nutrients, while essential for the growth and development of higher organisms, also serve as substrates for bacterial proliferation. For instance, organic matter plays a crucial role in supporting plant growth, enhancing soil fertility, and contributing to ecosystem stability. However, this same organic matter can become a double-edged sword; it provides an abundant source of energy and nutrients for bacteria, fostering their growth and potentially leading to overpopulation. This can result in shifts in microbial community dynamics, where '''pathogenic bacteria can thrive''', outcompeting beneficial species and disrupting the ecological balance. Thus, while organic matter is vital for the health of plants and ecosystems, its availability can inadvertently promote bacterial growth, presenting a complex interplay between nutrient availability and microbial behavior. | |||
<blockquote>"To speak about glutathione as either good or bad is scientifically incorrect; we must discuss ORP voltage and electromolecular charges instead. Our body requires oxidation continuously to survive, but in the right amount, just as a mobile phone needs 5V to charge. If we apply 50V, it will burn, just as our cells can be harmed by OH or superoxide radicals. This is not the case with oxygen, which is essential for life." | |||
</blockquote>Glutathione (GSH) plays a crucial role in maintaining cellular homeostasis and redox balance within the human body. Understanding its function requires a nuanced examination that goes beyond categorizing glutathione as simply “good” or “bad.” Instead, we must consider the broader context of oxidative-reduction potential (ORP) and the interplay of electromolecular charges in maintaining physiological equilibrium. | |||
The human body operates under a delicate balance of oxidation and reduction reactions that are vital for sustaining life. This balance can be analogized to the way electronic devices, such as mobile phones, require specific voltage levels for optimal operation. Just as a mobile phone typically requires around 5 volts to charge effectively, our cells also require a regulated amount of oxidative stress to function properly. Excessive oxidative stress, however, akin to applying 50 volts to a device designed for 5 volts, can lead to cellular damage and dysfunction. | |||
Oxidative stress '''arises from an imbalance''' between the production of reactive oxygen species (ROS) and the body’s ability to detoxify these reactive intermediates. While ROS play essential roles in cellular signaling and homeostasis, an '''overabundance''' can cause oxidative damage to lipids, proteins, and DNA. This is where the role of antioxidants, particularly glutathione, becomes significant. Glutathione serves as a key antioxidant that helps neutralize harmful radicals like hydroxyl radicals (•OH) and superoxide anions (O<sub>2</sub>•−), thereby protecting cellular integrity. | |||
Moreover, the concept of oxidative-reduction potential (ORP) is vital in understanding how various substances interact with ROS. For instance, chlorine dioxide (ClO<sub>2</sub>), which has an ORP of approximately 940 mV, exhibits potent antioxidant properties. This high ORP allows ClO<sub>2</sub> to act effectively against hydroxyl radicals and superoxide radicals. By donating electrons to these harmful species, ClO<sub>2</sub> helps mitigate oxidative stress, thereby enhancing cellular protection and maintaining redox balance. | |||
It is critical to recognize that oxygen itself is indispensable for life. It participates in aerobic respiration, facilitating ATP production through the mitochondrial electron transport chain. However, as a byproduct of this metabolic process, ROS are generated, necessitating robust antioxidant defenses to mitigate potential damage. Glutathione contributes significantly to this defense mechanism by participating in various enzymatic reactions and recycling other antioxidants, such as vitamins C and E. | |||
In summary, rather than labeling glutathione as simply beneficial or detrimental, it is imperative to appreciate its role within the complex network of redox biology. '''The body requires a balanced oxidative environment''' to thrive; thus, understanding ORP and electromolecular charges provides a more comprehensive framework for evaluating the function of glutathione in health and disease. Additionally, the role of substances like ClO<sub>2</sub> with high ORP values underscores the importance of considering various antioxidants in the context of redox equilibrium. It is this balance that enables our cells to harness the benefits of oxidation while safeguarding against its potential harms. | |||
The widespread belief that oxidation is inherently bad for our health and well-being is, in fact, scientifically misleading and fundamentally bogus. This misconception overlooks the complex nature of oxidation processes and their dual roles in biological systems. While it is true that excessive oxidative stress can lead to cellular damage and contribute to various diseases, it is essential to recognize that oxidation also plays a crucial role in many vital physiological functions. | |||
== Biochemical Processes Involved == | == Biochemical Processes Involved == |
Revision as of 10:48, 30 October 2024
Oxidants versus Antioxidants: Understanding Their Roles in Human Health
by Dr. h.c. Andreas Ludwig Kalcker
Introduction
Over the past fifty years, the widespread consumption of antioxidants has been strongly advocated as a critical and essential component of maintaining overall health and well-being. This article delves into and explores the foundational theories surrounding the concepts of oxidants and antioxidants, with particular focus on the influential free radical theory of aging that was introduced by Dr. Denham Harman back in 1956. According to this theory, free radicals, which are unstable molecules that can damage cellular components, play a significant role in the aging process and various diseases.
We thoroughly analyze the intricate biochemical mechanisms that underlie both oxidation and reduction processes, as well as the various roles played by reactive oxygen species (ROS). Research has shown that while ROS can contribute to oxidative stress, which is linked to conditions such as cancer, cardiovascular disease, and neurodegenerative disorders, they also play a crucial role in cell signaling and homeostasis. Additionally, we examine the implications of chlorine dioxide, considering it from the perspective of both an oxidant and an antioxidant. Scientific studies indicate that in low concentrations, chlorine dioxide can act as an antioxidant by modulating the oxidative stress response.
The interactions between these various substances are meticulously examined, with an emphasis on their significant roles in promoting cellular health and their diverse therapeutic applications in medical science. For instance, numerous clinical trials have demonstrated that certain antioxidants, like vitamin C and E, can reduce markers of oxidative stress in humans. Moreover, the ongoing research into phytochemicals such as flavonoids and polyphenols highlights their potential protective effects against oxidative damage and their ability to enhance overall health outcomes.
The relationship between oxidants and antioxidants has garnered considerable attention in biomedical research over several decades. The prevailing hypothesis posits that antioxidants can mitigate the detrimental effects of free radicals, specifically ROS. This paper aims to elucidate the mechanisms governing this relationship and evaluate its implications for health, with particular emphasis on treatments involving chlorine dioxide ( ClO2 in form of CDS).
The Free Radical Theory of Aging
The free radical theory of aging posits that oxidative damage, which is primarily inflicted by free radicals, plays a crucial role in contributing to the complex aging process and the onset of various diseases that are commonly associated with aging. According to Dr. Denham Harman's original formulation of this theory, hydroxyl radicals (OH) are particularly harmful as they can cause extensive and significant damage to cellular structures and components, which can ultimately lead to dysfunction, degeneration, and disease over time. Consequently, antioxidants, including well-known compounds like vitamin C, are believed to effectively counteract these damaging effects caused by free radicals. By neutralizing these harmful radicals, antioxidants provide a vital protective mechanism for cells against the detrimental impacts of oxidative stress, potentially slowing down the aging process and reducing the risk of age-related diseases.
Oxidation-Reduction Potential (ORP)
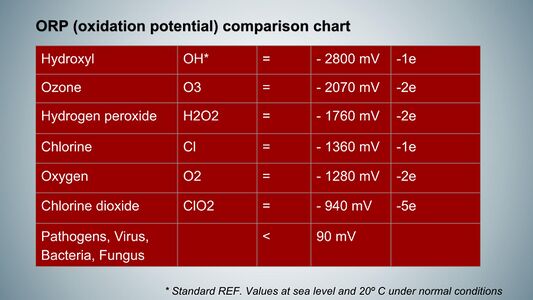
The oxidation-reduction potential (ORP) is a critical concept in biochemistry and environmental science, representing the ability of a chemical species to gain or lose electrons in redox (reduction-oxidation) reactions. Measured in volts (V) or millivolts (mV), ORP indicates the oxidative or reductive state of a solution, with a higher value signifying a greater tendency for a substance to act as an oxidizing agent, while a lower value indicates a stronger reducing agent. In human physiology, ORP typically ranges from 1.0 to 1.5 volts, reflecting the dynamic interplay between oxidants and antioxidants within cells. Oxidants, such as reactive oxygen species (ROS), are byproducts of normal cellular metabolism and play essential roles in cell signaling and immune responses; however, excessive oxidant production can lead to oxidative stress, damaging proteins, lipids, and DNA and contributing to diseases like cancer, cardiovascular disorders, and neurodegenerative conditions. Key antioxidants in the human body include Vitamin C, Vitamin E, and glutathione, which donate electrons to neutralize free radicals and prevent cellular damage. Maintaining an optimal ORP is essential for cellular integrity and function, influencing cellular signaling, metabolism, and immune function. ORP can be measured using specialized electrodes that provide real-time data about the redox state of biological fluids; for instance, elevated blood ORP is associated with various health conditions, including diabetes and cardiovascular diseases. Understanding ORP is vital for comprehending the balance between oxidants and antioxidants in biological systems, as this balance is crucial for overall cellular health and could lead to better strategies for preventing and managing diseases related to oxidative stress.
While other oxidizing agents such as ozone exhibit greater antiseptic properties in laboratory settings, their high oxidation potential of 2.07 V and brief half-life of approximately 15 minutes at 25 °C render them less effective for in vivo therapeutic applications. In contrast, chlorine dioxide (CDS) can provide between 4 to 5 electrons, enhancing its efficacy for in vivo applications.
Notably, many experts remain unaware that CDS possesses antioxidant properties that contribute to mitigating oxidative stress. These properties facilitate the neutralization of free radicals, thereby preserving cellular integrity.
The ORP value of chlorine dioxide (ClO₂) is recorded at 0.94 V, which is lower than the typical range observed in human cells (1.2 to 1.5 V). This suggests that under normal physiological conditions, chlorine dioxide is less aggressive and not harmful to human cells.
Conversely, hydroxyl radicals possess an ORP value of 2.8 V, indicating a strong oxidizing effect that can be detrimental to cellular health. Since chlorine dioxide exhibits a lower potential compared to hydroxyl radicals, it is capable of reducing these harmful species by accepting electrons from them, thereby neutralizing their oxidizing effects.
Regarding to concerns about chlorine dioxide's penetration into human cells, it's important to note that at the low concentrations used for health purposes, chlorine dioxide primarily acts as an oxidant in the extracellular environment. While it can potentially interact with cell membranes, its effects on intracellular components like DNA are not relevant at these very low concentrations due to the size of the human cell . Additionally, the body's natural antioxidant systems help mitigate any potential oxidative damage. The extent of oxidative damage depends on the oxidation-reduction potential (ORP) of the oxidant. Hydroxyl radicals (OH•) have a high ORP of 2800 mV, while chlorine dioxide has a relatively low ORP of 940 mV, even lower than oxygen (1360 mV). This means that chlorine dioxide operates at a voltage level compatible with human cells, which use oxygen for respiration. The antioxidant properties of chlorine dioxide at low concentrations are also attributed to its ORP being lower than highly reactive oxidants like hydroxyl radicals, allowing it to act as an antioxidant against them avoiding cellular damage due to excessive oxidative stress.
The reaction can be summarized as follows:

Hypochlorous acid (HClO), produced as an intermediate step through this reaction, plays a crucial role in immune response by combating bacteria, viruses, and other pathogens as well.
Glutathione
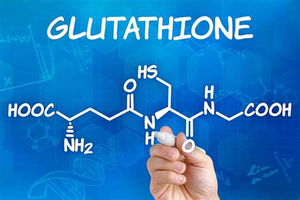
Glutathione, a crucial antioxidant found in human cells, plays a significant role in maintaining cellular health and protecting against oxidative stress. However, its availability is not limited to human physiology; bacteria also utilize glutathione to enhance their growth and proliferation. In microbial environments, glutathione acts as a key nutrient that facilitates various metabolic processes, enabling bacteria to thrive under the to high oxidative conditions Caused by OH radicals. While it is essential for the detoxification and repair mechanisms in human cells, the same properties allow bacteria to mitigate oxidative damage and promote their survival. This duality highlights the complex interplay between host and microbial populations, where glutathione serves as both a protective agent for human cells and a growth factor for bacteria. Understanding this relationship is vital for developing strategies to manage bacterial infections and maintain cellular integrity.
In the study of microbial ecology, one can observe that certain nutrients, while essential for the growth and development of higher organisms, also serve as substrates for bacterial proliferation. For instance, organic matter plays a crucial role in supporting plant growth, enhancing soil fertility, and contributing to ecosystem stability. However, this same organic matter can become a double-edged sword; it provides an abundant source of energy and nutrients for bacteria, fostering their growth and potentially leading to overpopulation. This can result in shifts in microbial community dynamics, where pathogenic bacteria can thrive, outcompeting beneficial species and disrupting the ecological balance. Thus, while organic matter is vital for the health of plants and ecosystems, its availability can inadvertently promote bacterial growth, presenting a complex interplay between nutrient availability and microbial behavior.
"To speak about glutathione as either good or bad is scientifically incorrect; we must discuss ORP voltage and electromolecular charges instead. Our body requires oxidation continuously to survive, but in the right amount, just as a mobile phone needs 5V to charge. If we apply 50V, it will burn, just as our cells can be harmed by OH or superoxide radicals. This is not the case with oxygen, which is essential for life."
Glutathione (GSH) plays a crucial role in maintaining cellular homeostasis and redox balance within the human body. Understanding its function requires a nuanced examination that goes beyond categorizing glutathione as simply “good” or “bad.” Instead, we must consider the broader context of oxidative-reduction potential (ORP) and the interplay of electromolecular charges in maintaining physiological equilibrium.
The human body operates under a delicate balance of oxidation and reduction reactions that are vital for sustaining life. This balance can be analogized to the way electronic devices, such as mobile phones, require specific voltage levels for optimal operation. Just as a mobile phone typically requires around 5 volts to charge effectively, our cells also require a regulated amount of oxidative stress to function properly. Excessive oxidative stress, however, akin to applying 50 volts to a device designed for 5 volts, can lead to cellular damage and dysfunction.
Oxidative stress arises from an imbalance between the production of reactive oxygen species (ROS) and the body’s ability to detoxify these reactive intermediates. While ROS play essential roles in cellular signaling and homeostasis, an overabundance can cause oxidative damage to lipids, proteins, and DNA. This is where the role of antioxidants, particularly glutathione, becomes significant. Glutathione serves as a key antioxidant that helps neutralize harmful radicals like hydroxyl radicals (•OH) and superoxide anions (O2•−), thereby protecting cellular integrity.
Moreover, the concept of oxidative-reduction potential (ORP) is vital in understanding how various substances interact with ROS. For instance, chlorine dioxide (ClO2), which has an ORP of approximately 940 mV, exhibits potent antioxidant properties. This high ORP allows ClO2 to act effectively against hydroxyl radicals and superoxide radicals. By donating electrons to these harmful species, ClO2 helps mitigate oxidative stress, thereby enhancing cellular protection and maintaining redox balance.
It is critical to recognize that oxygen itself is indispensable for life. It participates in aerobic respiration, facilitating ATP production through the mitochondrial electron transport chain. However, as a byproduct of this metabolic process, ROS are generated, necessitating robust antioxidant defenses to mitigate potential damage. Glutathione contributes significantly to this defense mechanism by participating in various enzymatic reactions and recycling other antioxidants, such as vitamins C and E.
In summary, rather than labeling glutathione as simply beneficial or detrimental, it is imperative to appreciate its role within the complex network of redox biology. The body requires a balanced oxidative environment to thrive; thus, understanding ORP and electromolecular charges provides a more comprehensive framework for evaluating the function of glutathione in health and disease. Additionally, the role of substances like ClO2 with high ORP values underscores the importance of considering various antioxidants in the context of redox equilibrium. It is this balance that enables our cells to harness the benefits of oxidation while safeguarding against its potential harms.
The widespread belief that oxidation is inherently bad for our health and well-being is, in fact, scientifically misleading and fundamentally bogus. This misconception overlooks the complex nature of oxidation processes and their dual roles in biological systems. While it is true that excessive oxidative stress can lead to cellular damage and contribute to various diseases, it is essential to recognize that oxidation also plays a crucial role in many vital physiological functions.
Biochemical Processes Involved
The process of ingesting food leads to the absorption of carbohydrates, which are primarily composed of various sugars, by our cells. Specifically, complex carbohydrates are broken down into monosaccharides like glucose, which is then utilized by cells as a primary energy source. Within these cells, there exist numerous organelles, among which mitochondria are considered paramount for the essential process of energy production. Mitochondria are often referred to as the "powerhouses" of the cell because they play a critical role in generating adenosine triphosphate (ATP) through a series of complex biochemical pathways known as oxidative phosphorylation and the citric acid cycle (Krebs cycle). These processes also produce important byproducts such as carbon dioxide (CO₂) and hydroxides (OH).
These byproducts are known to be highly reactive free radicals, with a significantly high oxidation-reduction potential (ORP) of approximately 2,800 millivolts. This high ORP indicates a strong tendency for these free radicals to react with other molecules, potentially leading to oxidative stress. Considering that typical cellular functions generally occur within a range of 1,000 to 1,500 millivolts, an excess of hydroxides generated within the cell necessitates the prompt action of antioxidants, such as glutathione and vitamin C, to effectively mitigate potential cellular damage and maintain overall cellular health.
Research has shown that oxidative stress is implicated in various diseases, including cancer, cardiovascular diseases, and neurodegenerative disorders. The balance between these reactive species and the antioxidant defenses is crucial for sustaining cellular integrity and function. Furthermore, studies have indicated that a diet rich in antioxidants can help enhance the body’s defense mechanisms against oxidative stress, highlighting the importance of nutrition in cellular health and longevity.
Release and Distribution of Chlorine Dioxide
Chlorine dioxide exhibits exceptional solubility in water due to its remarkably small molecular size and uniquely V-shaped molecular structure, which closely resembles that of water molecules. This specific configuration results in a spherical arrangement because of the molecular angle of 117.6°, in contrast to the 104.45° angle found in H₂O molecules. The unique geometrical arrangement of chlorine dioxide molecules, along with its electromolecular effects, likely contributes to the formation of hexagonal structures that have been observed in various chemical research studies. Research indicates that chlorine dioxide has a solubility of approximately 7.3 g/L at room temperature, which is significantly higher than many other chlorine compounds.
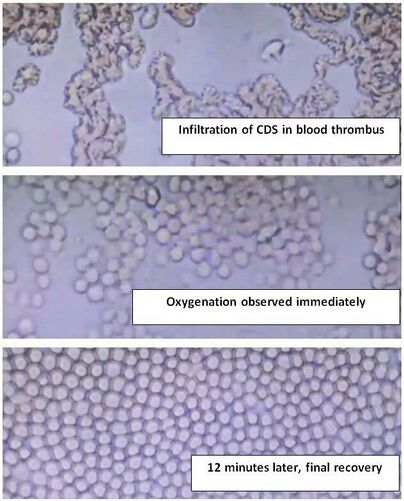
When chlorine dioxide is applied to deoxygenated blood and subsequently examined under a microscope, it is noted that erythrocytes, or red blood cells, arrange themselves homogeneously after a few minutes of exposure. This remarkable phenomenon may be attributed to an electron circulation that occurs within the hexagonal structure of the chlorine dioxide molecules, facilitating this orderly arrangement. Studies have shown that this interaction can influence erythrocyte morphology and functionality, potentially enhancing oxygen delivery to tissues. We observe an increase of the Z
Upon ingestion of a small quantity of CDS (chlorine dioxide solution) dissolved in water, the gas is released in the stomach through the process of evaporation at approximately 37.5 °C, which is the typical temperature of the human body. Notably, CDS begins to evaporate at much lower temperatures, reaching this point as low as 11 °C, in stark contrast to sodium chlorite, which does not evaporate until it reaches a significantly higher temperature of 170 °C. The absorption of CDS occurs directly through the gastric mucosa due to the high water content present in that tissue. Its similarity in size and structure to water molecules enables it to diffuse easily into interstitial spaces, following Fick's laws of gas diffusion, and rapidly distribute throughout all aqueous regions of the body.
The CDS molecule randomly distributes itself throughout the body due to its low solubility and small size, measuring approximately 160 nm, and it does so without undergoing hydrolysis. This distribution occurs according to Fick’s second law of mass conservation and takes place without any accompanying chemical reactions. This allows the chlorine dioxide molecules to cross the blood-brain barrier effectively, enabling their potential therapeutic applications in various medical contexts. Scientific studies have indicated that chlorine dioxide can exhibit antimicrobial properties, making it useful in disinfection and sterilization processes, as well as showing potential for treating certain medical conditions through its interaction with biological systems. Additionally, research has suggested that chlorine dioxide may have a role in modulating inflammatory responses, further expanding its relevance in clinical settings.
Oxygen Transport Capacities
The molecular weight of ClO₂ is 67 g/mol, whereas that of O₂ is 32 g/mol. Consequently, oxygen constitutes approximately 48% of the molecular weight of ClO₂; thus, 1 mg ClO₂ contains about 0.48 mg of oxygen:
- Thus, 1 ml CDS can release approximately 1.44 mg O₂, equating to 1 ml of dissolved O₂ in plasma corresponding to oxygen transport via 0.72 grams of hemoglobin at a partial pressure of 100%.
Consequently, following complete reaction over approximately two hours, 10 ml CDS can provide about 10 ml of oxygen molecules.
Importantly, chlorine dioxide binds oxygen without consuming it; the reaction occurs only when chlorine dioxide reaches problematic areas and dissociates due to an excess of protons. This is relevant for viral capsids (spikes) across various virus types; upon denaturation, spikes oxidize. In more acidic cellular environments, chlorine dioxide first reacts and dissociates, delivering oxygen to under-supplied mitochondria throughout the body. This process restores pH balance after eliminating existing pathogens or pH-acidic toxins while also enhancing cellular oxygen supply in these oxygen-deficient regions.
The Role of Chlorine Dioxide
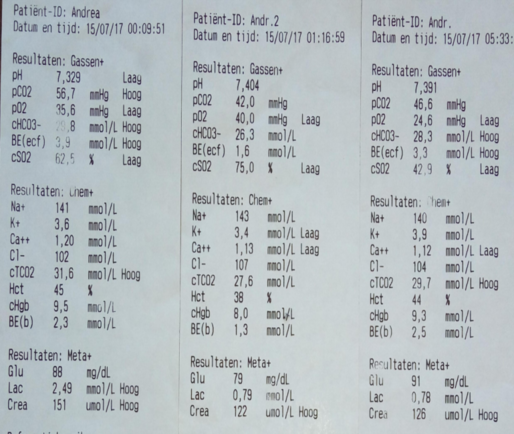
Chlorine dioxide has attracted considerable scholarly attention in recent years for its notable dual functionality as both an oxidant and an antioxidant, a characteristic that has significant implications across various fields of research and application. As an oxidant, chlorine dioxide operates effectively by targeting substances with an oxidation-reduction potential (ORP) lower than 940 millivolts, thus initiating the oxidation of these compounds. Specifically, microorganisms such as bacteria, which exhibit an ORP tolerance ranging from 50 to 90 millivolts, are particularly susceptible to oxidation when exposed to chlorine dioxide, rendering it a potent agent for microbial inactivation and control.
Crucially, one of the most salient features of chlorine dioxide is its relative safety concerning human cells; this is attributable to its lower ORP, which is significantly below the operating levels characteristic of human cellular environments. This differential in ORP underscores the selective reactivity of chlorine dioxide, allowing it to target pathogenic microorganisms without adversely affecting mammalian cells.
Moreover, chlorine dioxide demonstrates the capability to reduce hydroxides due to its inherent lower voltage and its ability to donate two electrons during redox reactions. This electron donation mechanism stands in stark contrast to conventional oxidation processes, which typically involve the theft of electrons from other molecular entities. Such properties not only elucidate the unique chemical behavior of chlorine dioxide but also underscore its potential utility in a variety of applications, including disinfection protocols and water treatment methodologies, where its oxidizing and reducing capabilities can be harnessed effectively to achieve desired outcomes in microbial control and chemical stabilization.
Therapeutic Benefits
Emerging studies in recent years indicate that chlorine dioxide can significantly enhance oxygen levels in the blood without negatively impacting overall health or contributing to adverse side effects. For example, after administering an intravenous infusion of 500 ml of a solution containing a ClO₂ concentration of 50 parts per million (ppm), measurable and remarkable increases in venous blood gases of up to 50% were observed among volunteers who participated in the study. In addition to intravenous administration, oral intake of chlorine dioxide also resulted in an average increase of around 30%, demonstrating its effectiveness through multiple delivery methods. Furthermore, significant decreases in lactate levels were recorded across various cases, which holds particular relevance for athletes who are susceptible to lactic acidosis following periods of intense physical exertion and strenuous workouts.
Additionally, chlorine dioxide has demonstrated notable improvements in renal function markers such as creatinine levels and also plays a role in the management of renal anemia. These findings strongly suggest its potential role in enhancing overall metabolic health and improving various physiological parameters that are crucial for optimal body function. This combination of benefits presents chlorine dioxide as a compound worthy of further exploration and consideration in both clinical and athletic settings.
Interactions Between Antioxidants and Chlorine Dioxide
The co-administration of antioxidants and chlorine dioxide presents an intriguing dynamic. When combined, these substances may react with one another, potentially nullifying their individual effects. Therefore, it is recommended that antioxidants be avoided during chlorine dioxide treatments to prevent the neutralization of its therapeutic effects. Specifically, strong antioxidants such as vitamin C or citrus juices should not be consumed concurrently with chlorine dioxide.
Understanding this interaction is essential for optimizing therapeutic strategies and ensuring effective treatment outcomes.
Conclusion
In conclusion, the intricate balance between oxidants and antioxidants plays a vital role in human health. While antioxidants such as vitamin C are essential for combating oxidative stress, their interaction with substances like chlorine dioxide necessitates careful consideration in treatment protocols. Further research is warranted to explore the complexities of these interactions and their implications for health manage